This interview article about ITER was conducted in May 2020 and has been edited for brevity and clarity.
About Tim Luce
Tim Luce works as Chief Scientist at ITER. He has a PhD in Astrophysical Sciences from Princeton University. He worked with the DIII-D Tokamak at General Atomics in the US for 30 years before he joined ITER in 2017.
About ITER
ITER is an international collaboration project in southern France, involving 35 nations that aims to build the world’s largest fusion energy device. It will be the first magnetic fusion device to produce net energy (more energy output than input). ITER’s goal is not to build a commercial fusion power plant, but instead a blueprint of a functioning system that will eventually be used by the involved countries to develop their own power plants.
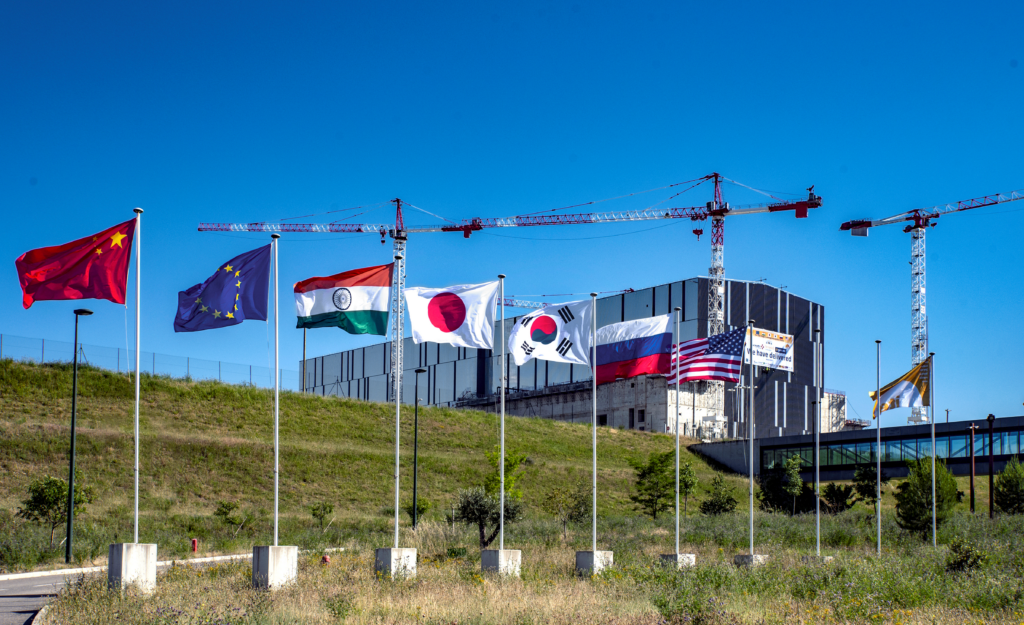
Credit © ITER Organization, http://www.iter.org/
Could you please describe the principle of fusion?
– If you think of the periodic table of elements, there are very heavy elements like uranium and plutonium and there are very light elements like hydrogen. Their weight has to do with the number of particles in the nucleus. Just like Goldilocks, everything wants to be in the middle of the periodic table; everything wants to be nickel basically. If you take heavy elements and break them or if you take light elements and put them together, there is excess energy. For fusion, we are putting together two of the lightest elements – two different types of hydrogen called deuterium and tritium.
– What you get from that is a large amount of energy and it comes out in two forms. One is a neutron, which will have 80% of the energy. The neutron is neutral in terms of electrical charge, which means it will go through our magnetic field directly into the walls where it gets absorbed and heats a fluid. After that it’s just like any kind of power plant that yields a thermal energy source, drives turbines and create electricity. Then you also get 20% of the energy which comes out as a helium nucleus, which is called an alpha particle. Because it has charge, it will slow down in the plasma due to the magnetic field and its energy will heat the plasma.
“The main disadvantage with fusion energy is that it is hard. We haven’t done it yet”
What are the pros and cons of this energy source?
– First of all, the fuel is greatly abundant. We can get deuterium out of any water source, so it is universally available. Also, one gram fusion fuel yields the energy of eight tonnes of fossil fuel. We are working with the strongest of the four forces of nature, which is holding together the nucleus. This means that when we liberate energy from the strong force we get much more yield for the same mass of fuel that relies on for example the electromagnetic force or the weak force.
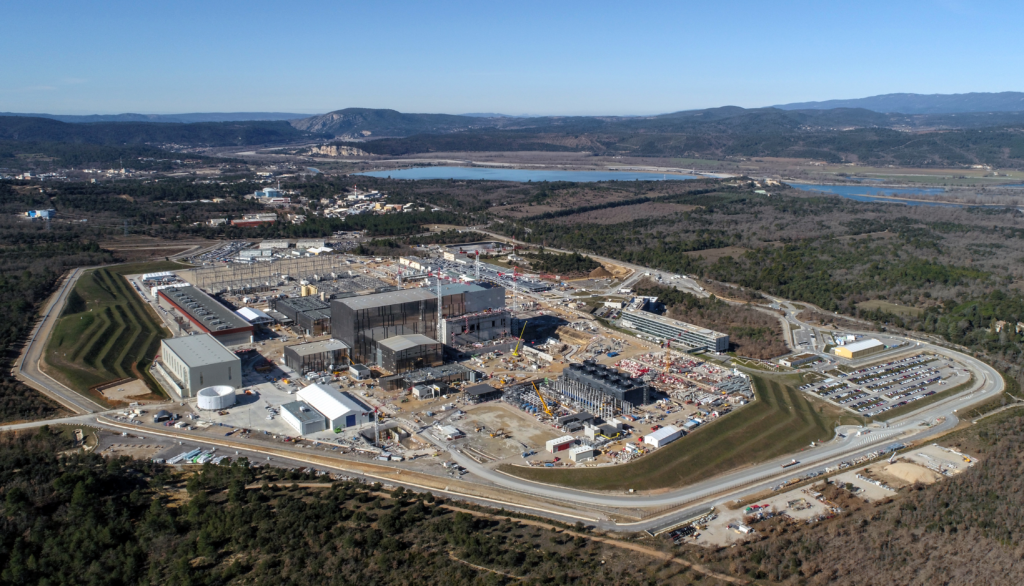
Credit © ITER Organization/EJF Riche, http://www.iter.org/
– There are several advantages compared to fission, which is the principle of today’s nuclear power plants. Fission plants need to be re-fueled annually or every two years, which is not the case with fusion. If something goes wrong in a fusion reaction, it immediately stops, which eliminates the risk of a run-away reaction and a potential accident that you have to consider with fission. There is also much less radioactive waste in a fusion power plant, the waste is not water soluble and the decay times are much shorter.
– We are talking about decay times of around 100-200 years. You can imagine having something taken care of for a hundred years, but what about a by-product that might have tens of thousands or even hundred of thousands of years life time, as is the case with fission? Add to that the fact that the by-product of fission is water soluble, so you have to worry about it going into groundwater. The main disadvantage with fusion energy is that it is hard. We haven’t done it yet, and that is essentially the mission of ITER – to bring fusion to demonstration at the power plant scale and prove that it can be done with the tokamak concept.
The second part of the fuel is tritium. How do you acquire that?
– Tritium has a decay time of 12.3 years, so it doesn’t occur in nature. But with the fusion power plant that we envision, it can have a closed fuel cycle. This means that it can generate its own tritium fuel from the reactions without compromising the electrical output. That is really the objective. You do need a certain amount of tritium to get up on the step, but once you’re there you can have a completely closed cycle. From the proliferation point of view that’s good since you don’t need to bring fuel in and out of the site; it’s all there. Tritium isn’t an enormous proliferation risk but it is a nuclear controlled substance, so we need to pay attention to how it’s used and how it’s transported.
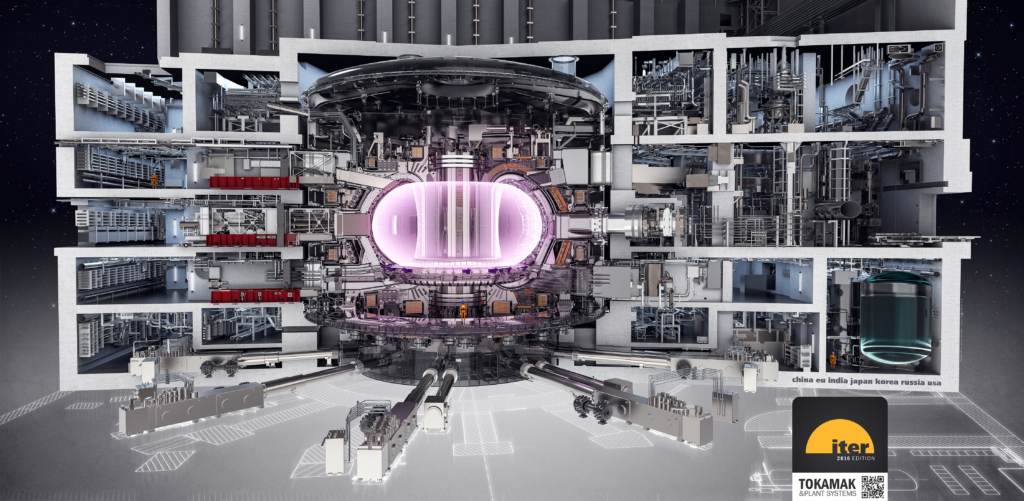
Credit © ITER Organization, http://www.iter.org/
What is Q?
The objective of ITER is Q = 10, with the definition of Q as the ratio of the fusion power output to the power necessary input. At 10 it means that we’re getting 10 times the energy out than we’re putting in. The highest value of Q reached so far is less than 1, which means we still haven’t seen a case where you get more power out than what you put in. I believe the highest official value is from the JET Tokamak in the UK, which reached a Q of about 0.7.
What are the main challenges that are laying ahead? Is there a clear plan of everything that needs to be done or are there problems that are still not solved?
– We have a very clear idea of what we believe will happen in ITER. There’s a lot of scientific literature about predictions of what ITER will do. Of course, predictions are only worth the paper they’re written on; you have to go and do it. There will undoubtedly be surprises and challenges and we have a research plan that addresses those progressively.
– There are two main scientific questions or challenges: First, we need a temperature at the center above a hundred million degrees Celsius for the fusion reactions to go. We need a vacuum at absolute zero outside the edge of the plasma, and the plasma is only 2 meters across. This means we have an enormous temperature gradient which requires insulation in some sense to be maintained. The magnetic field strength basically sets the quality of the insulation.
“from the scientific point of view, that’s where the fun is; to be an explorer. From an investor or a government point of view, you like certainty.”
– Second is the self-heating challenge. To get to a Q of 10 you can’t simply keep putting in power. You need self-heating power from the fusion reactions themselves to sustain the temperature necessary for the reactions. We have very extensive and quite detailed first principle calculations of what we expect will happen. But plasmas are quite fascinating objects that tend to surprise us. We have to go and do it and from the scientific point of view, that’s where the fun is; to be an explorer. From an investor or a government point of view, you like certainty.
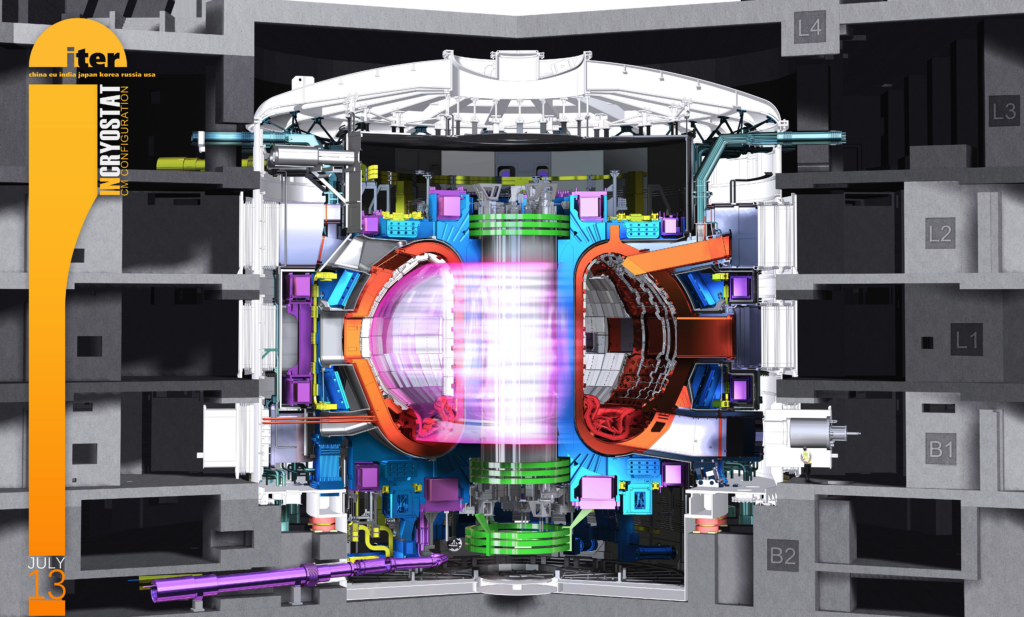
Credit © ITER Organization, http://www.iter.org/
Considering the immense advantages of this technology, are you surprised that there isn’t a race to reach a functioning power plant first? Or is that still too far away in time for it to be an attractive investment?
– My personal view is that the main problem with fusion is that the buy-in unit cost is high. You can put solar cells on your house that might be able to actually power your home for somewhere between 10 and 30 thousand euros. You need substantially more than that to invest in a single fusion device that could produce a thousand megawatts of energy. The return of investment for an industry, a real investor, is too long at this point. Governments have various time scales at which they value things.
– What happened with ITER is that rather than individual countries investing, they’ve chosen to reduce their own initial investment by teaming together, They could afford to go by a faster time plan but they’ve chosen to go this way. The ITER agreement gives them full intellectual property generated by the project. Also, 85% of what’s on the site is brought by what’s called in-kind, meaning that each of those members actually invests in their own industry and in their own countries.
What’s the current status of the project and what are the big milestones laying ahead?
– It’s great timing actually, because yesterday we completed the first significant event of assembling the tokamak. The magnets are superconducting, so they have to be held at 4 degrees above absolute zero, -269 °C, which means it has to be all in a giant thermos bottle, a cryostat. Just yesterday we placed the base of the cryostat inside the tokamak building, which is the first act of assembling the whole tokamak itself. That was quite exciting!
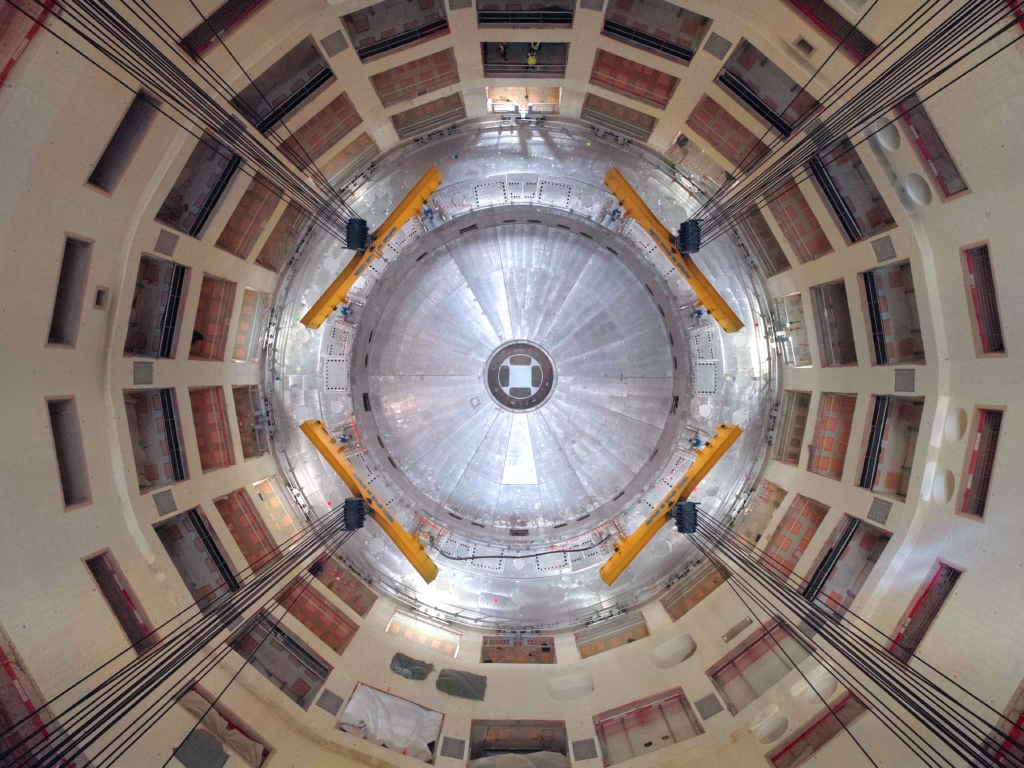
Credit © ITER Organization/EJF Riche, http://www.iter.org/
– From here, the schedule is for completion of all of the tokamak and all of the necessary supporting systems to operate a very simple, basic plasma by the end of 2024. We will spend a year making those systems work together, passing the regulatory hurdles, and then make our first plasma by the end of 2025. Following that, we continue to assemble things that we have to put inside the tokamak to protect the walls and also bring the other heating systems into service. At the end of 2035 we plan on getting final regulatory approval to introduce the nuclear fuel. From that point, we will work quite rapidly for achieving the project objectives, the gain of 10, with 500 MW fusion power for more than 300 seconds. We’re projecting that the objectives will take between 4-6 years to achieve once we’ve introduced the nuclear fuel.
When can we expect the first fusion power plant to deliver electricity?
– The vision is that each of the ITER members will go off to make the next step, which is a demonstration power plant. It was chosen in ITER not to put in the electricity generating capability, both because it is conventional technology and we wouldn’t pay back the cost of doing it, so it didn’t make sense. There are also challenges in the demonstration power plant that have to do with materials that can take continuous exposure. We’re really only starting the technological solutions at ITER, for example, we’re starting to look at closing the fuel cycle but we won’t generate our own fuel at all. We’re starting to look at the exposure to neutrons, the exposure to high temperature plasma of materials, but we know that the materials that we’ve chosen now to achieve our goals aren’t really the best for fusion energy power plants. The success of ITER will be achieving the physics objectives and showing that it’s performing as expected even before we introduce the nuclear fuel.
If you found this article interesting, you should probably also check these out:
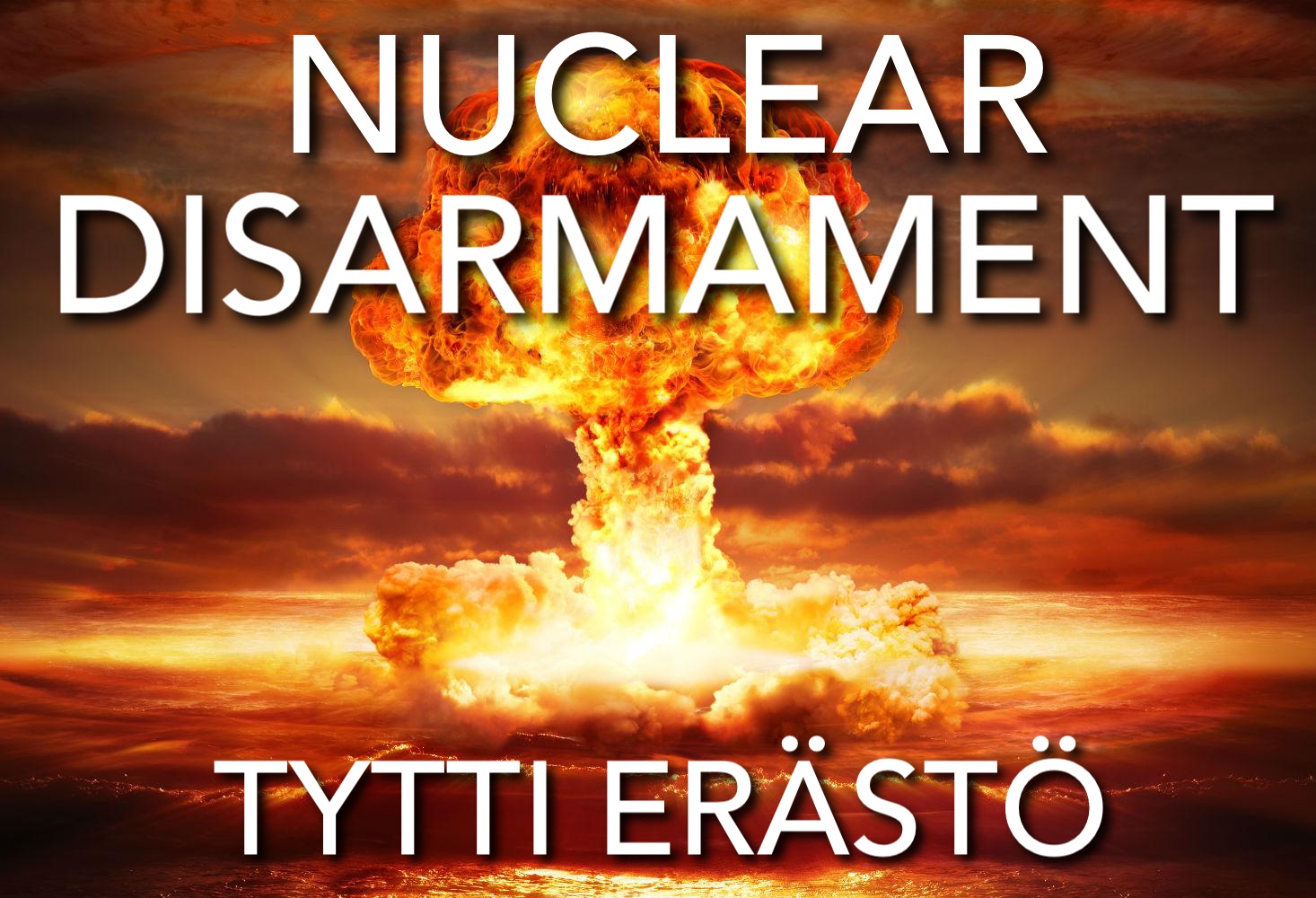
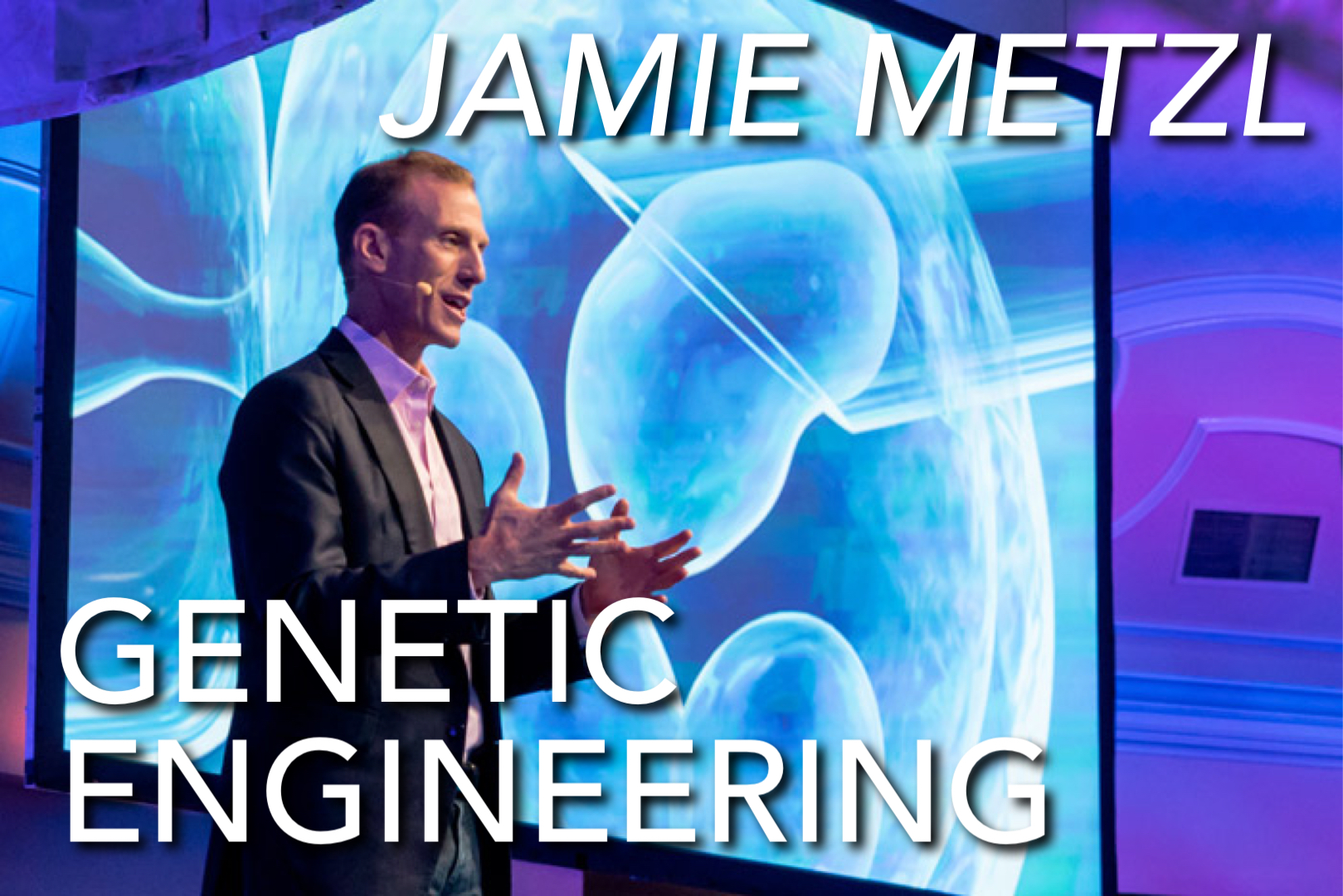